Batteries
Batteries
3D batteries
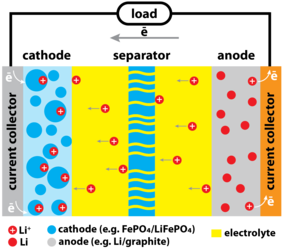
Battery is a device that stores energy to later be converted into electricity using chemical reactions. Rechargeable lithium ion batteries (LIBs) due to their overall superior performance in terms of energy density and power efficiency dominate the market for consumer electronics, wearables and automotive applications. Global Li ion battery industry demonstrates a spectacular continuous growth and in a decade is expected to surpass the current records of 120 GWh (€24 billion) with a ten-fold increase.1Technological development, however, lags this increasing demand. Although a tremendous progress has been made in the LIBs’ characteristics on the active materials side, which has been recently acknowledged by a Nobel Prize in chemistry, the performance of LIBs is growing incrementally by only several percent every year.2 This significantly hinders further technological progress in all areas, where batteries with higher power, shorter charging times and larger capacities are needed.
LIBs operation is based on the insertion and de-insertion of lithium at the electrodes and transport of lithium ions from an anode to a cathode. The transport of lithium occurs through an electrolyte, which provides a conductive medium for Li+ ions, while electrons flow through an external circuit. In a typical configuration (see Figure 1), LIB consists of metallic current collectors, coated with the active electrode material, capable for reversible intercalation and deintercalation of lithium, such as lithium metal oxides, LiFePO4 and others at the cathode and graphite, lithium titanate, transition metals that form alloys with lithium and other materials at the anode. The electrodes are usually separated by a porous polymeric membrane that prevents direct electrical contact between the electrodes.
The current paradigm in Li ion technology is based on the two-dimensional planar configuration of battery cells. The major drawback of this layout is that it is impossible to achieve simultaneously high output power (current) and high energy density (capacity) of the battery. Typically, the increase of the energy density requires an extension of the thickness of active electrode layers, which comes at a cost of reduced power due to slower transport rates of ions through thicker films and higher ohmic resistances during battery operation, related to low conductivity of cathode materials and to chemical gradients that arise upon redistribution of ionic species. Since similar issues (and some others) arise during charging, batteries require relatively long charging times that approach tens of minutes and hours because higher rates usually lead to a capacity loss. For example, a typical time to reach 80% capacity of the Tesla car battery is around 30 minutes,3 same for the iPhone for its 50% state of charge.4 The disadvantages of LIBs related to long charging times impose the need to search for materials with higher energy density to reach higher capacity that will allow extending the range of electric vehicles and improving operation times of electronic devices, which are currently limited.
In contrast to the mainstream in battery research, we are working on an unconventional approach with the aim to make a major leap in charging rates (to restore power within seconds) and introduce LIBs capable to deliver high output current without compromising other performance characteristics and with the use of already established electrode materials. This has a potential to change the paradigm not only in battery engineering but also establish new future for mobile electronics and electric vehicles.
While it is impossible to achieve such a groundbreaking improvement with the standard design of battery cells, this project will use concepts of additive manufacturing (AM) for fabrication of closely spaced high aspect ratio nanostructures forming the anode and the cathode. From the simple geometric calculations one can thus anticipate 698 to 806-fold increase in the electrode surface area. Advantages of 3D design immediately translate into: (i) higher overall current enabled by larger surface area, providing an equivalent increase in power; (ii) faster ion transport due to nanoscale spacing between electrodes, and also faster charging allowed by very low current density, enabling almost three orders of magnitude higher charging rates with the no adverse effects on capacity; (iii) improved heat dissipation and reduced heating (low Ohmic losses). To illustrate this advancement, one can imagine charging a mobile phone battery to its 50% capacity in 2.6 seconds (!), which is nearly instantaneous.
The intrinsic advantage of the 3D battery layout is nanoscale separation between the battery electrodes and high overall surface area, which does not compromise other performance characteristics. To demonstrate that this design doesn’t affect the gravimetric capacity, one can consider batteries with typical high-performance cathode materials, like LiFePO4 (theoretical specific capacity 170 A h kg-1) and Li4Ti5O12 (175 A h kg-1). Comparison of a 3D battery with pillars on a square grid, containing FePO4 in the form of thin film (45 nm) covering the iron pillar core, to the “flat” battery of the same dimensions (total cell thickness of 100 μm, with cathode and the anode of 40 μm) suggests that gravimetric capacity remains almost unaffected (84.8 vs 88.8 A h kg-1), although some reduction is expected in volumetric characteristics (A h L-1).
Meanwhile current research efforts worldwide are almost exclusively focused on materials’ performance, our group is exploring new 3D printing approaches to make a breakthrough in the battery technology.
References
1. Tsiropoulos, I.; Tarvydas, D.; Lebedeva, N. Li-ion Batteries for Mobility and Stationary Storage Applications. JRC Science for Policy Report 2018.
2. Yoshino, A. Development of the Lithium-Ion Battery and Recent Technological Trends. In Lithium-Ion Batteries; Pistoia, G., Ed.; Elsevier: Amsterdam, 2014; pp 1-20.
3. Tesla Cars website. www.tesla.com/supercharger (accessed 07 October 2019).
4. Apple website. support.apple.com/en-us/HT208137 (accessed 07 October 2019).