The DyNano Project: Dynamics at the Nanoscale
The DyNano Project: Dynamics at the Nanoscale
DyNano - Dynamics at the Nanoscale bundles the activities in the field of nano- , quantum- and energy research at the Faculty V- Mathematics and Natural Sciences of the Carl von Ossietzky University Oldenburg. DyNano (Dynamics at the Nanoscale) is a collaborative research programm of the Ministry of Research and Culture of Lower Saxony to fund our activities with an overall volume of > 2 Mio €.
The research focus is on the development of novel experimental methods for the investigation of quantum dynamics in nanoscale solids as well as in molecules and molecular aggregates. With this work, we aim to gain a detailed understanding of dynamical processes in nanostructures and open up new ways to control energy transport on small length scales.
As a collaborative platform, DyNano aims to
- bring together the scientific expertise and research techniques available in the individual working groups in order to address larger and more complex research questions across groups.
- to promote the exchange of knowledge between the groups and to initiate new collaborations.
- Link scientific research expertise across disciplines and form new, interdisciplinary networks.
- to bundle scientific infrastructure and use it effectively and in a way that can be financed sustainably.
Kick-off meeting of the collaborative project DyNano!
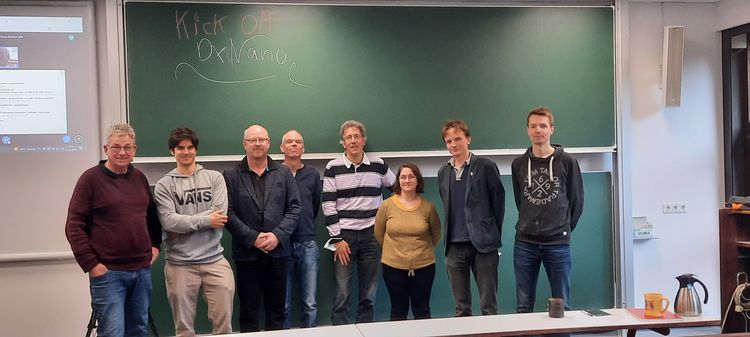
Our DyNano seminar in WS 2024/2025 at W2-1-148
Date | Speaker | Title |
06/11/2024 | Wei Wang | Advances in scattering SNOM for nanoscale optical characterization |
13/11/2024 | Sergei Tretiak / Los Alamos | To be announced |
04/12/2024 | Franziska Maria Kühling, Oldenburg University | Fundamentals of bipolar electrochemistry and its possible application in optical and electrochemical characterisation of single plasmonic nanostructures |
13/12/2024 Special date | Prof. Dr. Anne L'Huillier, Universität Lund | Attosecond pulses of light for the study of electron dynamics |
18/12/2024 | Prof. Michael Bauer, Kiel University | Time-resolved Photoelectron Spectroscopy |
15/01/2025 | Jan Vogelsang, Oldenburg University | The Oldenburg Attosecond PEEM: Assembly and first experiments |
22/01/2025 | Alexander Simon, Oldenburg University | Phase-dependent reactivity studies of copper vanadate systems |
Our DyNano Seminar for the Summer Semester 2024:
Date | Speaker | Title |
15/05 | Talat S. Rahman, University of Central Florida | Excited-state charge dynamics in 2D materials: Interplay of excitons and phonons |
Special date 27/05 | Katharina Kaiser, Göttingen | Time-resolved Scanning tunnelling microscopy |
05/06 | Jens Drawer | |
19/06 | Marcel Reutzel, Göttingen | Momentum microscopy & 2D materials: Excitons in space and time |
03/07 | Lars Englert, Oldenburg | Photoelectron spectroscopy with high harmonics radiation |
At the Institute of Physics, the following research groups and junior research groups are involved in DyNano - Dynamics at the Nanoscale:
PD Dr Svend-Age Biehs | Theoretical Nano-Optics | Institute of Physics |
Prof. Dr. Caterina Cocchi | Theoretical Solid State Physics | Institute of Physics |
Institute of Physics | ||
Prof. Dr. Christoph Lienau | Ultrafast Nanooptics | Institute of Physics |
Scanning Probe Spectroscopy | Institute of Physics | |
Prof. Dr. Ilia A. Solov'yov | Quantum Biology and Computational Physics | Institute of Physics |
Prof. Dr. Christian Schneider | Quantum Materials | Institute of Physics |
Dr. Jan Vogelsang | Attosecond Nano-Optics | Institute of Physics |
Prof. Dr. Michael Wark | Photocatalysis and Charge Carrier Dynamics | Institute of Chemistry |
Prof. Dr. Gunther Wittstock | Electrochemistry and Interfaces of Condensed Systems | Institute of Chemistry |
Institute of Physics |
A1. Coherent light-matter coupling and ultrafast dynamics of exciton polaritons in atomically thin films
Schneider, Lienau
Project A1 addresses the central question of the spatial and temporal scales on which coherence builds up in strongly coupled light-matter systems, and how this affects energy transfer. Atomically thin TMDCs belong to one of the most interesting classes of materials in modern photonics: excitons in TMDC single layers have huge oscillator strength, as well as extraordinarily interesting topological properties, giving rise to manifold perspectives both in the field of coherent light-matter coupling and energy and charge carrier transport.
We therefore investigate the formation, coherence, and dynamics of polaritons in the regime of strong light-matter interaction between excitons in a TMDC single layer and the optical resonance of a high Q microcavity. Here, we use a recently developed transportable open cavity (see Fig.). Complementary, we consider the coupling of excitons to plasmonic gratings with ultrasmall mode volumes fabricated by focused ion beam lithography from single-crystal gold flakes. Due to the strong coupling to the light field, new quasiparticles - exciton polaritons - unlike pure excitons, propagate over many µm in TMDC structures. This propagation can be ballistic or diffusive. First, the spatial propagation of the polaritons at different quasiparticle densities, temperatures, and beyond the threshold for polariton condensation is investigated , using spatially resolved interferometry to determine the coherence properties in the condensed regime.
The coherent dynamics of the system will be analyzed using polarization-controlled 2DES. This is relevant because coherent Rabi oscillations on the fs scale are expressed in the regime of strong light-matter coupling, which have not been previously observed in TMDC microcavities. The use of 2DES on strongly coupled TMDC resonator structures will provide important insights into how the influence of the particular valley topology, as well as the intrinsic nonlinearity of the excitons in TMDC layers, manifests itself on the time scale of the coherent Rabi oscillations and how it affects strong coupling and entanglement between excitons and photons/plasmons.
B1. Controlling ultrafast dynamics through conical intersections by supercontinuum polarization shaping
De Sio, Wollenhaupt
The aim of this project is to explore new concepts for the control of nanoscale energy transport in organic donor-acceptor materials. Using two-dimensional electronic spectroscopy (2DES) we could recently observe for the first time that intermolecular conical intersections (CoIns) govern the light-induced, sub-50 fs energy relaxation dynamics in organic molecular aggregates used as photoactive materials in organic electronics (1). CoIns are ubiquitous in molecules but were not yet observed in solids. Their discovery opens up interesting new prospects for the control of ultrafast energy and charge transport dynamics in organic functional materials, for example by coupling the materials to external light modes. Through controlled light-induced modifications of the potential energy surface, this approach opens up new opportunities for the control of ultrafast energy relaxation and transport dynamics in organic nanostructures with potential implications for energy technologies.
To this end, we combine the complementary expertise of the PIs in 2DES (1-4), supercontinuum polarization shaping (5) and photoelectron tomography (6-8). Using polarization-tailored femtosecond excitation pulses, we aim at manipulating the direction and speed with which the optically launched wave packet approaches the CoIn. While optical 2DES probes the dynamics on optically bright potential energy surfaces, photoelectrons provide access to the ensuing dynamics on optically dark states. To validate the photoelectron tomography method, we will perform reference experiments on model molecules in the gas phase and investigate the signatures of a nuclear wave packet traversing a CoIn in the three-dimensional photoelectron momentum distribution (6,7). The combination of all-optical and photoelectron detection methods with polarization shaping holds great promise for a comprehensive understanding of the ultrafast nonadiabatic dynamics through CoIns and for their control. Our studies will contribute to the development of future organic optoelectronic devices with tailored nanoscale energy transport.
(1) A. De Sio et al, Nature Nanotechnology 16, 63-68 (2021)
(2) X.T. Nguyen et al, The Journal of Physical Chemistry Letters 10, 5414-5421 (2019)
(3) A. De Sio, C. Lienau, Physical Chemistry Chemical Physics 19, 18813-18830 (2017)
(4) A. De Sio et al., Nature Communications 7, 13742 (2016)
(5) S. Kerbstadt et al, Optics Express 25, 12518 (2017)
(6) D. Pengel et al., Physical Review Letters 118, 053003 (2017)
(7) S. Kerbstadt et al, Nature Communications 10, 658 (2019)
(8) K. Eickhoff et al., Physical Review A 104, 052805 (2021) (Editor’s Suggestion)
B2. Exploring Fundamental Photo-catalytic Processes in Copper-Vanadate Model Systems at the Nanoscale
Nilius, Wark
Photo-catalysis is expected to evolve to a main technology for sustainable production of commodity chemicals and green energy in the future. Despite a large number of experiments, the fundamental principles of photoactive processes are not yet understood at the atomic scale. This concerns, in particular, the influence of local structural and stoichiometric inhomogeneities, such as line defects, interfaces and grain boundaries, on the diffusion, live time and recombination probability of the photo-induced carriers. Typically, the necessary experiments are hindered by the large structural and electronic complexity of photo-active materials that cannot be accessed by common microscopic and spectroscopic techniques.
Aim of this project is to overcome this ‘structure gap’ by a combination of high-resolution physical and chemical methods. For this purpose, two model systems will be prepared, (i) crystalline V-doped cuprous oxide films grown by physical vapor deposition and (ii) copper-vanadate powder samples produced by hydrothermal and sol-gel synthesis. The atomic structure of the resulting mixed oxides will be probed either by electron diffraction and scanning tunneling microscopy for the thin-film samples, or by X-ray diffraction and electron microscopy for the powder materials. The chemical and optical character of both samples will be accessed by photoelectron and NMR spectroscopy, as well as UV-Vis, absorption and luminescence spectroscopy, respectively. Whereas the macroscopic activity of the powder samples, e.g. in photocatalytic water splitting, is probed with a batch reactor, the reactivity of the crystalline films is determined locally by monitoring photo-driven charge transfer into suitable adsorbates bound to the surface. For this purpose, molecules with high electron (W3O9) and hole affinity (methyl viologen) are placed and optically activated at the surface. Changes in the molecular conformation due to photo-driven charge transfer can now be probed at the single-molecule level directly by STM imaging. In addition, the morphology and local optical activity of the samples can be correlated by means of STM luminescence spectroscopy, opening a pathway to determine the impact of grain boundaries and exciton traps on the photo-induced charge generation. By applying physical and chemical methods onto similar model systems, a better understanding of photocatalytic reactions and a substantial decrease of the ‘structure gap’ are expected from this project.
B3. Atomic modelling of ultrafast charge transfer in donor-acceptor complexes in different environments.
Cocchi, Solov’yov
In this project, ultrafast charge-transfer dynamics is studied in laser-excited organic donor/acceptor complexes that interact with various environments, such as liquid solutions, electromagnetic cavities, shielding substrates, and plasmonic nanostructures. Our goal is to uncover the basic mechanisms on the femto- to nanosecond scale, and to understand how the charge-transfer process is influenced by the interaction with their environment. Atomistic simulations are used, combining a quantum mechanical treatment of the photoactive system and a classical description of the environment and the incident time-dependent field. Time-dependent density functional theory is used to describe the dynamics in the sub-ps time window, coupled with the Ehrenfest scheme for treating electron-vibrational coupling. This approach is supplemented by quantum and molecular mechanical simulations, in which the charge-transfer process is described mechanistically under the influence of the molecular environment with the help of quantum mechanics.
B4. Theoretical description of energy transfer on the nanoscale
Solov’yov, Biehs, Cocchi
By interacting with a plasmonic environment, entanglement and long-range energy transfer and near-field heat transfer between two nanoparticles can be enhanced and the processes greatly accelerated compared to isolated structures. The relevant time scales range from fs-ns and the length scales from nm to μm. By combining numerical methods, including density functional theory (DFT) and molecular dynamics (MD) and the semi-analytical Green's function methods (GFM), the project aims to reach a new level of understanding of energy transfer through near-field interactions. The focus is on the ultrafast heat transport between two nanoparticles in the extreme near-field regime in which phonon tunneling dominates. This highly topical and controversial topic represents an excellent testing ground to compare our methods and to take first steps towards atomistic modeling of STM and SNOM experiments.
To analyze the ultrafast near-field heat transfer between two nanoparticles, two SiO2 nanoparticles are modeled using a combination of MD and GFM approaches. This allows to study the transition from photon to phonon tunneling dominated RHT. On the basis of DFT calculations, optimal materials are sought to observe strong and long-range phonon tunneling. MD and GFM are also used to elucidate the underlying ultrafast dynamics of heat flow and its fluctuations in the transition from the macro to the micro scale. The coupling of the microscopic systems to a plasmonic environment is investigated using a combination of MD/DFT and GFM. Specifically, we plan to model the RHT between particles and the photonic density of states of a nanoparticle, a molecule, or a monolayer structure in the vicinity of a substrate.
C1. STM/SNOM Hybrid Microscope for a Simultaneous Detection of the Electronic and Optical Density of States in Nanostructures
Nilius, Lienau
Aim of the project is the combination of two main concepts in experimental nano-optics that is electron-induced luminescence spectroscopy in a scanning tunneling microscope (LSTM) and resonant nearfield scattering spectroscopy (s-SNOM). The new hybrid microscope thus opens various experimental pathways to explore many-particle phenomena at the nanoscale. The s-SNOM technique is based on a broadband spectral excitation of nano-objects, followed by an amplitude and phase analysis of the resonantly scattered light in the far-field. The LSTM technique, on the other hand, exploits the controlled occupation of electronic states by electron tunneling from the STM tip and probes their radiative decay via far-field photon detection. Both approaches deliver complementary information on the optical and electronic properties of surfaces. While the LSTM probes single-particle excitations in the electronic density of states, the s-SNOM addresses plasmonic and excitonic modes, i.e., many-particle interactions in presence of Coulomb- and correlation effects. From comparing measurements conducted on the same nano-object, fascinating, new insights into many-particle interactions at the nanoscale are expected.
Goal of the project is the construction of a low-temperature scanning probe microscope with several optical input and output channels. Several operation modes can be realized with the new setup, for example topographic imaging at the atomic level, elastic tunneling spectroscopy, electron-induced luminescence and interferometric scattering spectroscopy as well as Raman spectroscopy in the tip-sample contact. Initially, the instrument will be employed to probe the exciton response of transition metal dichalcogenides (TMDCs), putting emphasis on the role of their chemical identity, defect landscape and stacking sequence. From the experiments, new insights into the fundamental interplay of morphology, excitonic properties and spatial-temporal carrier transport at the nanoscale are expected.
C3. Attosecond Streaking in Optical Near Fields
Vogelsang, Wollenhaupt
Excitation of bulk semiconductors such as silicon or zinc oxide with strong light fields leads to transient metallization of the material within a few femtoseconds (1, 2). Despite the importance of this process for future light-driven electronics, it has not yet been investigated for application-relevant nanostructures. Only by nanostructuring the material will it be possible to keep the photon numbers required for the switching process sufficiently low and to minimize heat losses. The aim of this project is to make strong, transient optical fields in the vicinity of nanostructures directly visible and thus to follow this metallization process directly. Experiments to characterize the XUV beamline apply polarization and phase modulated white light to generate high harmonics XUV laser pulses. We use a velocity map imaging spectrometer to measure the angle resolved energy distribution of photoelectrons originating from the interaction of XUV pulses with atoms. The next step advances towards nanostructures. Here, photoelectrons are emitted from inner shells by attosecond XUV pulses and then accelerated in the optical near-field of the nanostructure. In this process, a time-delayed visible pulse (3) is used to generate the optical near field in a VIS pump XUV probe experiment (Fig. 1). The photoelectron spectra (4) allow a direct analysis of the time dynamics of the optical near field (5) and the metallization process.
This will elucidate for the first time the influence of nanoscopically inhomogeneous field distributions on optically excited band structure changes in connection with local transport processes (6).
-
L. Gierster, S. Vempati, J. Stahler, Ultrafast generation and decay of a surface metal. Nature Communications 12, 978 (2021).
-
M. Schultze, K. Ramasesha, C. D. Pemmaraju, S. A. Sato, D. Whitmore, A. Gandman, J. S. Prell, L. J. Borja, D. Prendergast, K. Yabana, D. M. Neumark, S. R. Leone, Attosecond band-gap dynamics in silicon. Science 346, 1348 (2014).
-
S. Kerbstadt, D. Timmer, L. Englert, T. Bayer, M. Wollenhaupt, Ultrashort polarization-tailored bichromatic fields from a CEP-stable white light supercontinuum. Optics Express 25, 12518 (2017).
-
Y. C. Cheng, S. Mikaelsson, S. Nandi, L. Ramisch, C. Guo, S. Carlstrom, A. Harth, J. Vogelsang, M. Miranda, C. L. Arnold, A. Lhuillier, M. Gisselbrecht, Controlling photoionization using attosecond time-slit interferences. Proceedings of the National Academy of Sciences of the United States of America 117, 10727 (2020).
-
B. Förg et al., Attosecond nanoscale near-field sampling. Nature Communications 7, 11717 (2016).
-
L. Wittenbecher, E. V. Bostrom, J. Vogelsang, S. Lehman, K. A. Dick, C. Verdozzi, D. Zigmantas, A. Mikkelsen, Unraveling the Ultrafast Hot Electron Dynamics in Semiconductor Nanowires. ACS Nano 15, 1133 (2021).