Contact
Mailing Address
Visitors
Polarization Modulation Infrared Reflection Absorption Spectroscopy (PM IRRAS)
Spectroscopic or microscopic techniques have to be used to probe structural changes in molecular assemblies adsorbed on solid surfaces. Spectroscopic techniques operate by irradiation of the surface with electromagnetic radiation and collecting the reflected or transmitted radiation. Vibrational spectroscopy probes gas, liquid and solid phases with in the infrared wavelength range. Infrared spectroscopy (IRS) is particularly attractive for the structural analysis of molecular assemblies at interfaces because:
- It allows identification of the molecular structure and conformation of complex molecules (e.g., biopolymers, macromolecules);
- Different functional groups absorb the IR light at different wavelength allowing for simultaneous analysis of each kind of species present at the interface;
- A complex sample composition does not limit the spectral resolution and sensitivity of IRS;
- No labeling is required;
- Reflection-based techniques are applicable for studies of material adsorbed at solid interfaces.
To probe in situ structural and conformation changes in molecular films at solid surfaces (e.g., electrodes), we use polarization modulation infrared reflection absorption spectroscopy (PM IRRAS).
Instrumentation
Vertex-70 (Bruker) with external reflection unit (Bruler), polarisation modulator and electrochemical cells for this setup.
Fundamental of the Technique
Greenler demonstrated that the intensity of the electric field vector of an IR beam reflected from a metal surface (e.g., gold) depends on the polarization of the incoming radiation. A constructive interference occurs for p-polarized light at the gold surface by which the electric filed perpendicular to the surface is enhanced (Figure 2a, 3). The reflected IR radiation experiences a slight decrease in intensity, due to the absorption of the light by species adsorbed at the gold surface, carrying information about the composition of the interface. The s-polarized light undergoes a destructive interference and its electric field vector, which is parallel to the sample surface, has zero intensity at the gold|air interface (Figure 2b, 3). Therefore, the s-polarized light is not able to interact with species present at the interface, but would still cause absorption along the beam path (in the atmosphere of the spectrometer and in the solution above the elctrode). It can therefore serves are a reference spectrum that includes all absorption except for the molecules directly attached to the sample surface.
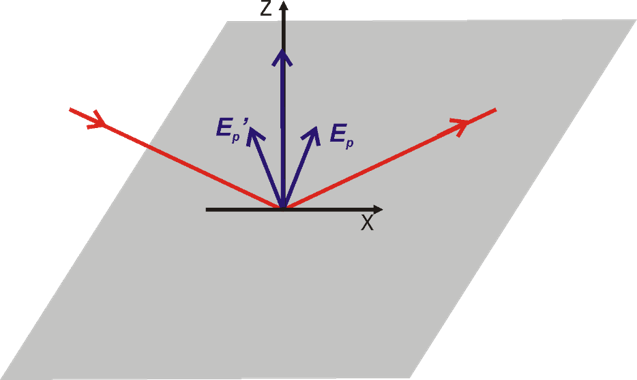
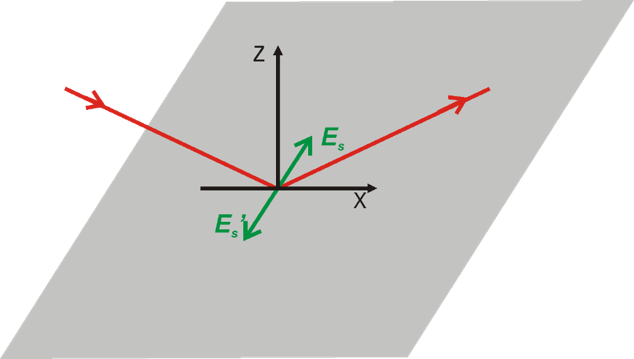
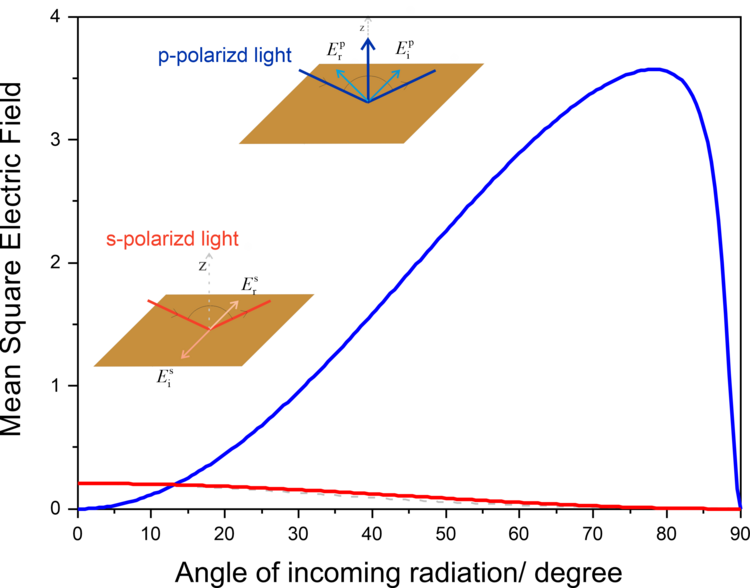
Greenler provided the theoretical bases for the development of infrared reflection-absorption spectroscopy (IRRAS). However, the absorption of thin molecular films present at interfaces is very low (in the range of 10-3 – 10-5 absorption units). In addition, the environmental background (H2O vapor and CO2) are not fully compensated by measuring the reference spectrum.
In 1979 Hipps and Crosby showed that a fast modulation of the polarization state of the incoming linearly polarized electromagnetic radiation, during the recording of IRS measurements gives a double modulated differential reflectance spectrum. It has a huge advantage of the cancellation of a strong background signal relative to the absorption of the molecules at the interface. A few years later, PM IRRAS was introduced to study the composition, structure and orientation of organic molecules adsorbed adsorbed on metallic surfaces. Thanks to an excellent signal-to-noise ratio, this technique was quickly adopted to study monolayers at water|air and solid|water interfaces. The mirrors for IR radiation are metallic surfaces (Au, Pt, Ag or Cu). These materials conduct electric current and are commonly used in electrochemical studies.
The interface between the electrode material and the electrolyte solution is in focus of electrochemical studies. PM IRRAS provides excellent possibilities to analyze potential-driven changes in the structure of molecular adsorbates in situ. This applies to changes in conformation, orientation and solvation of molecular films at electrode surfaces. Over the last years, we gained experience in studies of redox-inactive and redox-active mono- and multilayers of small organic and metalloorganic molecules, ionic liquids as well as films of macromolecules such as polymers, protein or DNA strands.
Models of biological cell membranes are an important research object for us. Lipids and membrane-associated proteins have anisotropic orientation with a clearly distinguishable stratified structure shown in Figure 4a. Due to the fact that lipids and proteins absorb IR light at different frequencies (e.g., CH stretching modes in acyl chains or C=O stretching modes in lipids and proteins) simultaneous analysis is possible for each kind of species present in the membrane on the gold surface. Thus, a supramolecular assembly of a model membrane shown in Figure 4a can be studied with a sub-molecular resolution as illustrated in Figure 4b.
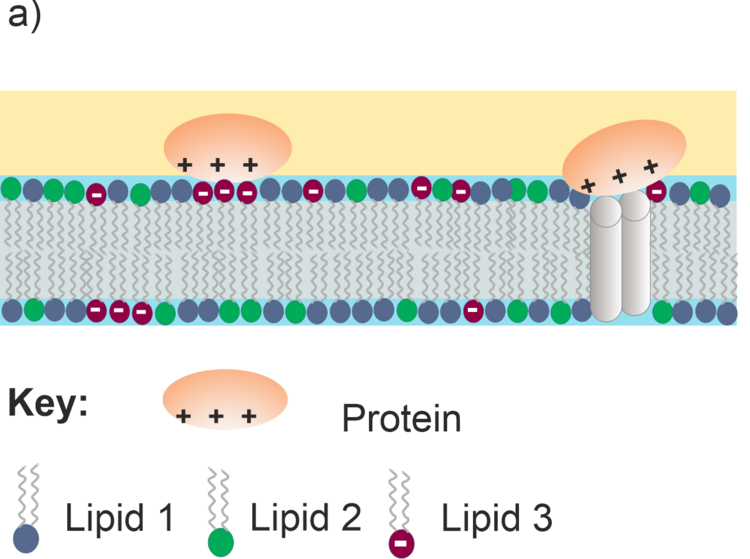
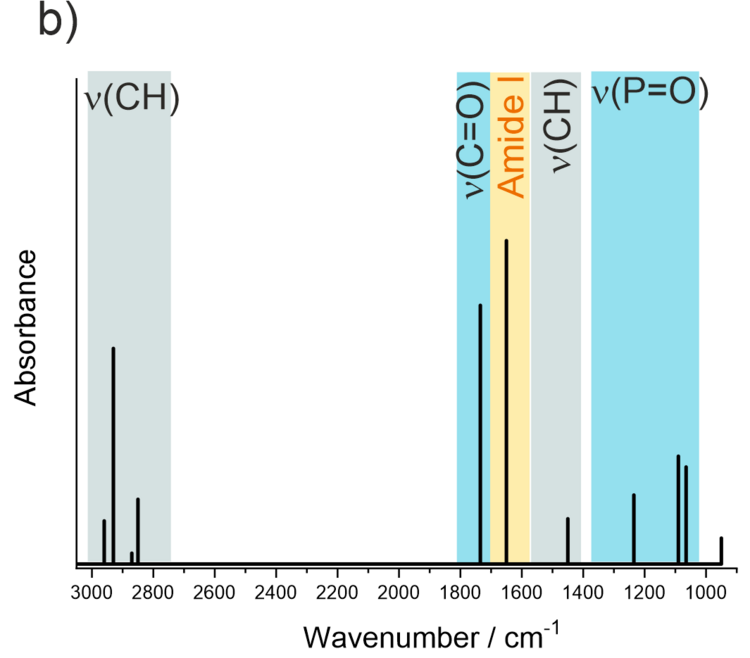
Figure 4. a) Schematic structure of a multicomponent lipid bilayer with an interacting protein; b) schematic IR spectrum showing absorption modes of different molecular fragments in the lipid-protein supramolecular assembly.
Orientation Analysis of Ordered Adsorbate Layers
Interestingly, the relative intensities of IR absorption modes in an anisotropic film may significantly differ from the spectra measured in a bulk phase. Figure 5a shows IR transmission spectrum of lipid vesicles in D2O and of a lipid bilayer adsorbed on the gold surface.
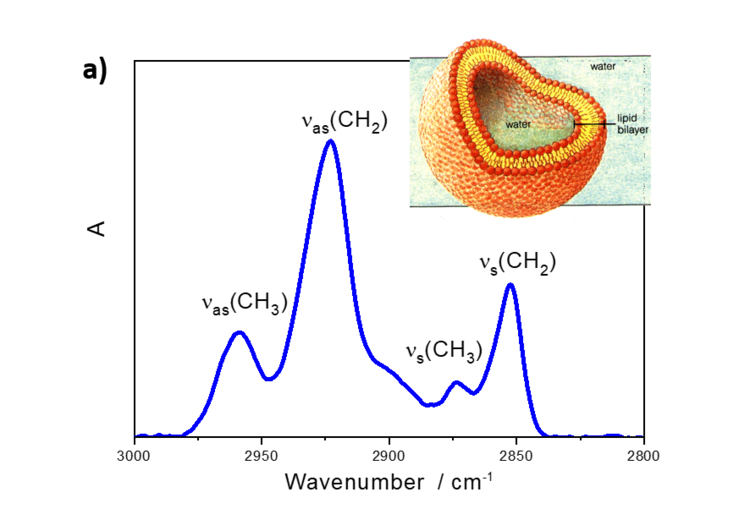
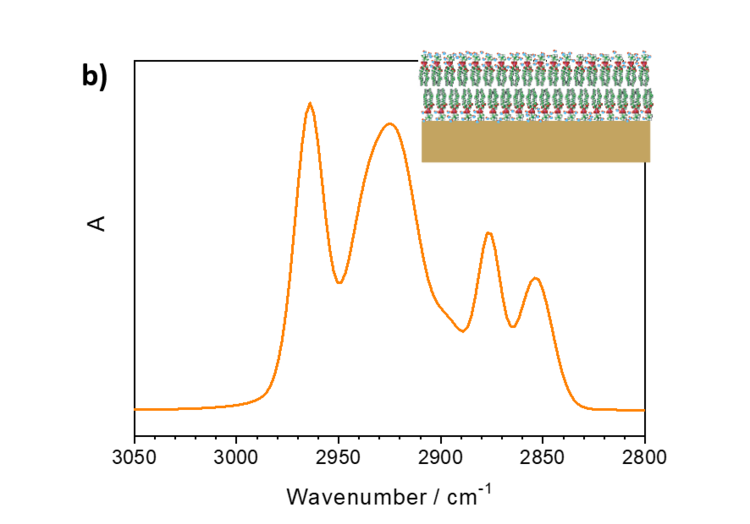
Figure 5. a) IR transmission spectrum in the CH stretching modes region of DMPC vesicles in D2O and b) PM IRRA spectrum of the DMPC bilayer on the Au surface.
DMPC is a phospholipoid molecule containing two saturated myristoyl (C14) chains. One DMPC molecule contains 24 CH2 and two CH3 groups. Both methylene and methyl groups have comparable extinction coefficients. As expected, the CH2 stretching modes in DMPC vesicles have significantly higher intensities than the CH3 stretching modes. In the bilayer however, the methyl and methylene stretching modes have comparable intensities.
These spectral changes are due to the surface selection rule of IRRAS and are used to determine average orientation of different molecular fragments (e.g., hydrocarbon chains) in anisotropic films. The integral intensity of a given IR absorption mode is given by equation 1:
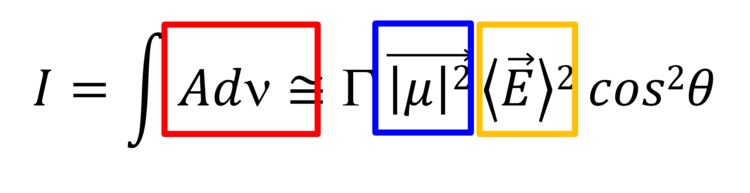
Equation (1)
where Gamma is the surface concentration of the adsorbed species, µ is the transition dipole vector of a given mode, E is the electric field vector of the electromagnetic radiation and theta is the angle between these two vectors.
Thus, to calculate the theta angle, the following steps have to be done:
- Determination of the integral intensity of a given IR absorption mode. In the case of overlapped modes: deconvolution of the IR absorption modes (determination of the second derivative: number of IR absorption modes, deconvolution using Gaussian and Lorenz functions)
- Knowledge of the orientation of the transition dipole vector in of a given IR vibration
- The electric field vector is normal to the surface in PM IRRAS.
Thus, attenuation of the intensities of the methylene stretching modes in the DMPC bilayer is connected to the well-defined orientation of the myristoyl chains in the membrane. Figure 6 illustrates this situation and shows exemplary orientations of a hydrocarbon chain fragment of an amphiphilic molecule adsorbed on a solid surface.
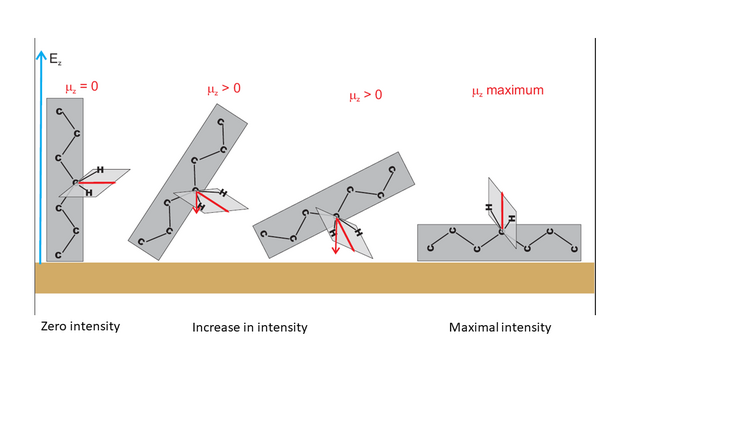
Figure 6 shows that the theta angle corresponds to the average orientation of the transition dipole moment vector versus the surface normal. When these two vectors are normal to each other, the integral intensity of this IR absorption mode will decrease to zero. Tilting of the hydrocarbon chain will cause a gradual increase in the intensity of this mode, until a maximum value for the parallel orientation of both vectors is reached.
Note that the theta angle cannot be determined directly from the PM IRRA spectrum. A reference system is required. This reference system represents a PM IRRA spectrum of randomly oriented molecules in the film of a given thickness. This spectrum can be calculated from the transmission solution spectrum of the studied molecule, e.g., from lipid vesicles. In an isotropic (randomly oriented) film, the average theta angle between the two vectors equals 53.54 ° (magic angle). Knowing the integral intensities of the IR absorption mode form PM IRRAS experiments and for the random film the theta angle can be calculated from equation 2,
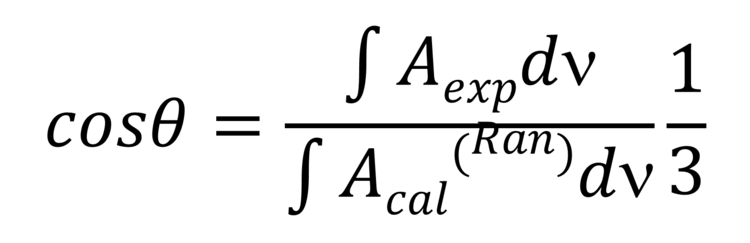
(Equation 2)
Application of PM IRRAS
PM IRRAS is used for qualitative and quantitative analysis. It allows us to identify molecules present at the interface and identify products of electrochemical reactions. The selective enhancement of IR absorption modes provides the basis to analyze quantitatively the orientation of different functional groups in anisotropic films. We have profound experience in studies of
- the structure of asymmetric lipid membranes of mammals and microbes
- conformational changes accompanying lipid-protein interactions
- potential-driven changes in conformation and stability of DNA double helices, their interaction with small zwitterionic molecules and enzymes
- electrical double layer of ionic liquids
- potential driven changes in monolayers of redox-active metallosurfactants with rectifying properties
- structure, conformation and composition of redox-active polymer films
- potential-dependent misfolding of protein films
- corrosion of light metals such as magnesium or aluminum.