Activity Imaging of Immobilized Enzymes
Contact
Mailing Address
Visitors
Activity Imaging of Immobilized Enzymes
Binding of Proteins to Surfaces
For some fundamental studies but also for applications in sensors, biochemical functional carriers such as enzymes or antibodies need to be attached (immobilized) to surfaces while maintaining their special properties. In many cases, there is also an interest in converting the activity or binding events on these proteins into an electrical or optical signal. For this purpose, signal converters (transducers) are used
We focus on the generation of electrical signals for the course of catalytic (enzymatic) reactions. In this case the transducer is an electrode. This can happen, for example, when products of the enzymatic reaction are oxidized or reduced at an electrode. To ensure that as many as possible of the particles generated by the enzyme also reach the electrode, the biomolecules must be immobilized at a dense distance from the electrode. The interfaces for immobilization and electrochemical conversion can be identical (Figure 1), intertwined (Figure 2) or spatially separated (Figure 3).
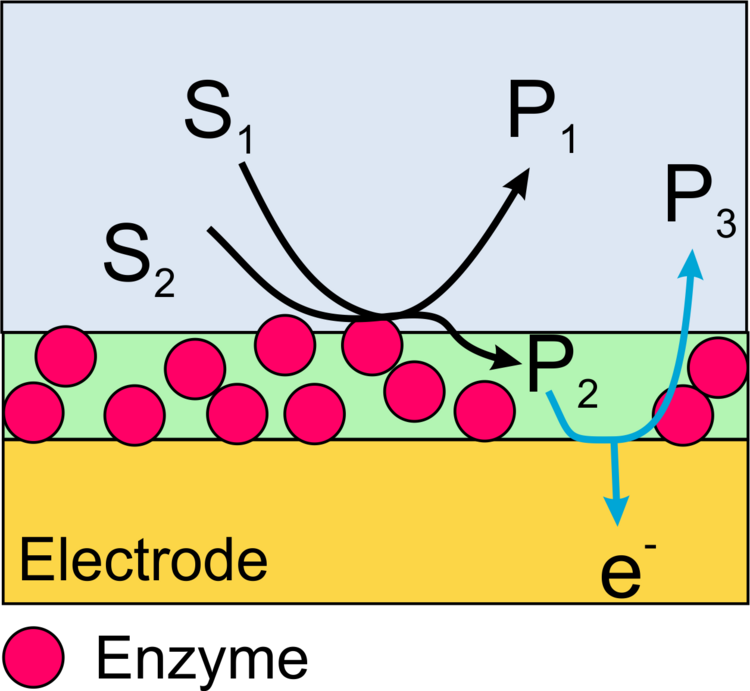
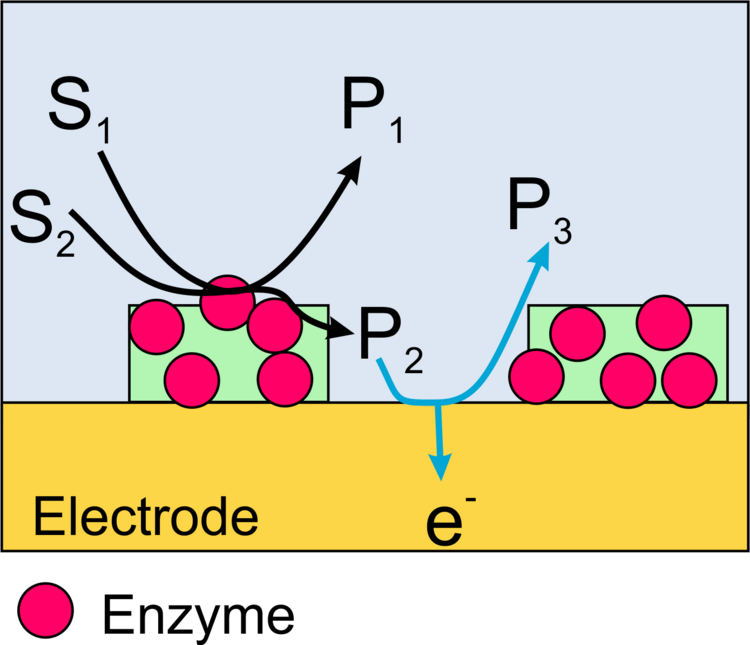
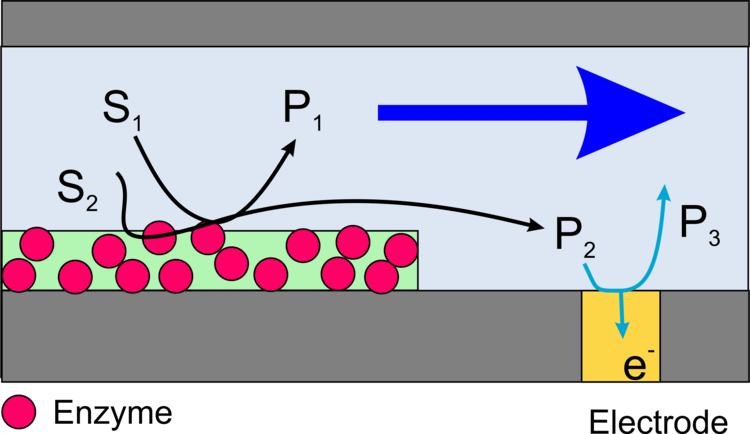
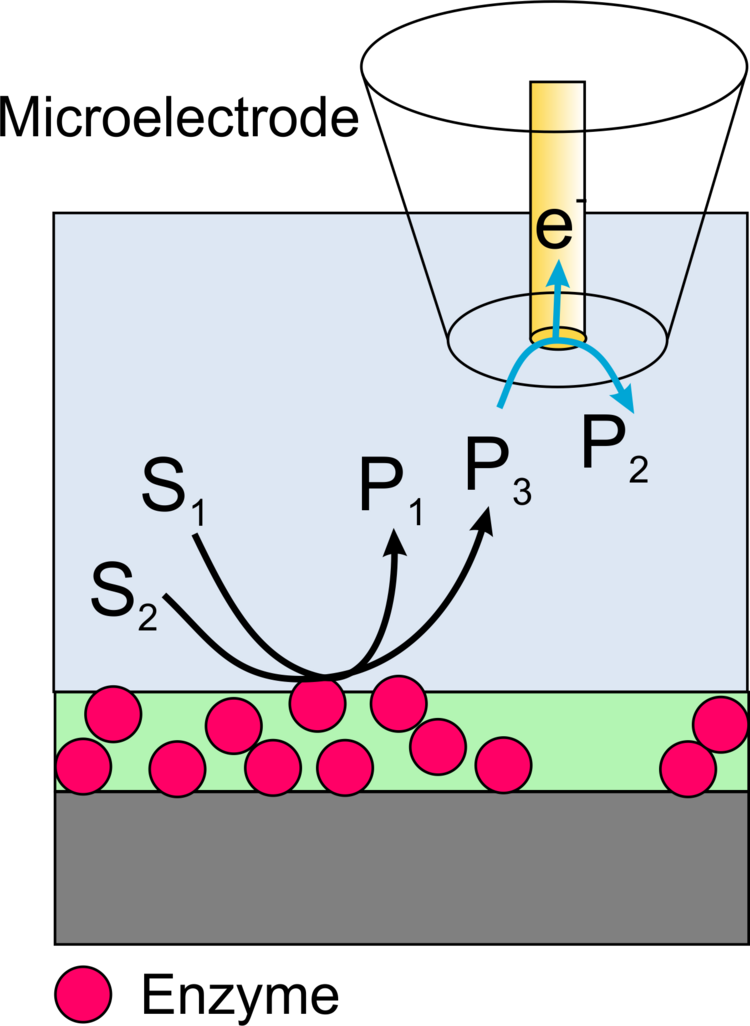
Figure 3. Schematic cross-section of a surface in which the carrier and transducer surfaces are spatially separated; a) in a microfluidic channel array; b) in a scanning electrochemical microscope.
Functional Coupling of Enzymatic Reactions with Electrodes
Electron transfer at the transducer surface can occur via oxidation of a reaction product of the enzymatic reaction (Figure 4) or via electrochemical regeneration of a redox mediator (Redoxmediator, Figure 5) or in special cases via direct electron transfer between electrode and protein (Figure 6).
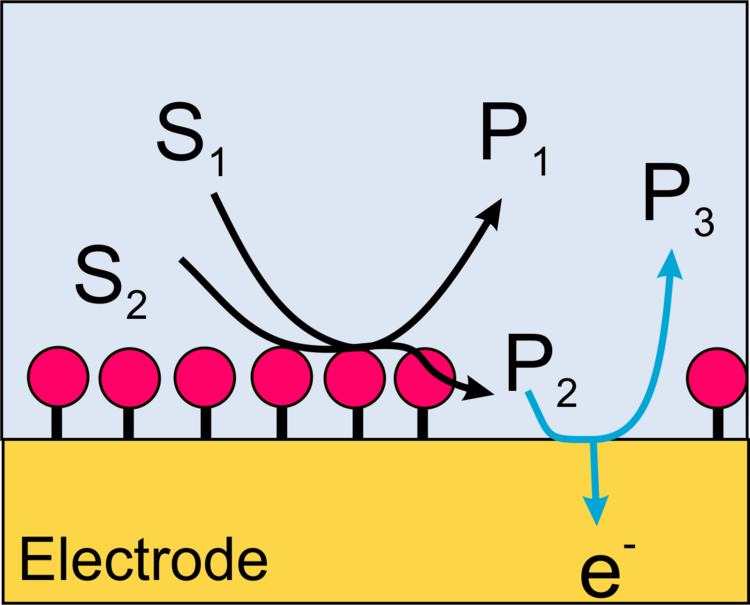

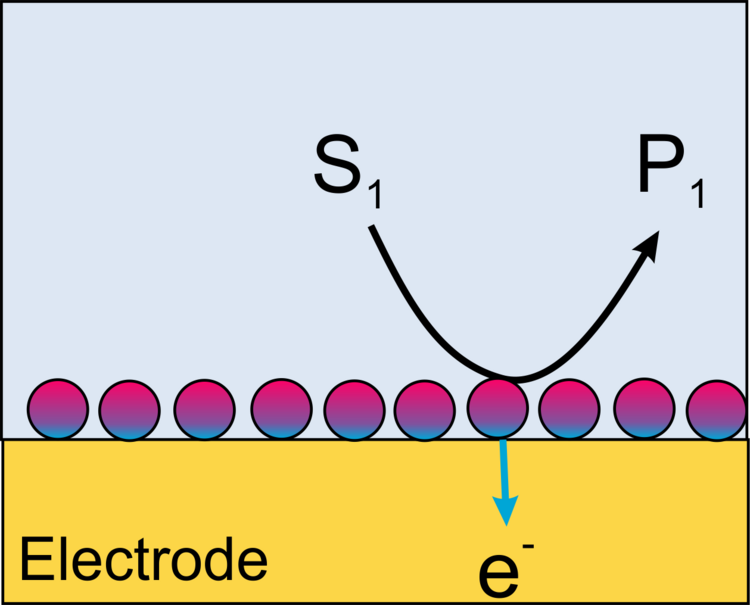
Molecular Design of Interfaces
Depending on which transduction principles are to be used, different supramolecular interface architectures can be considered (Figures 7-12).
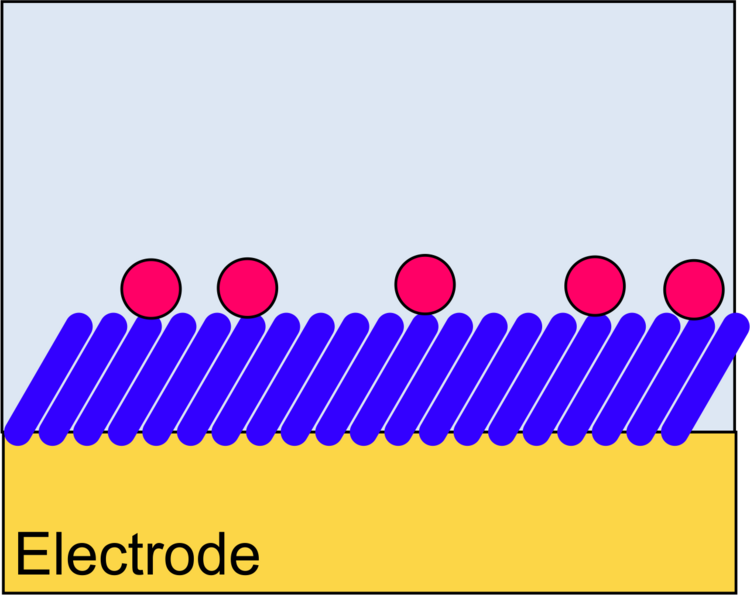
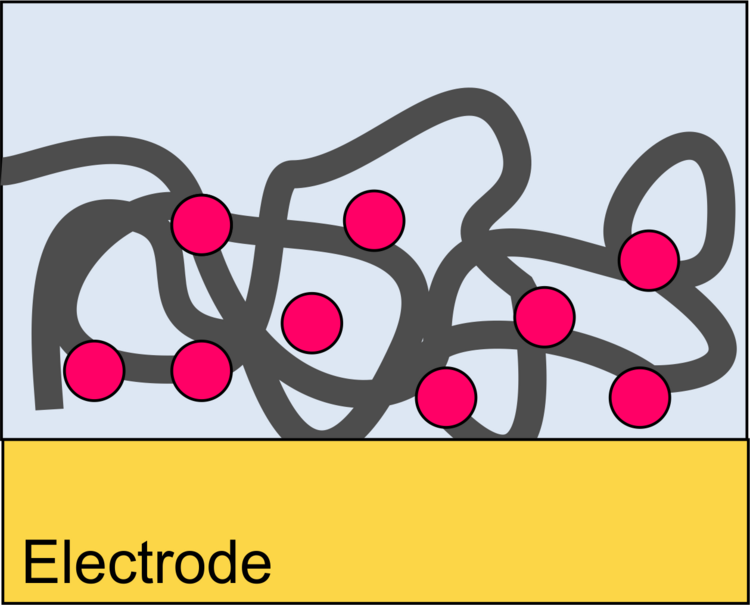
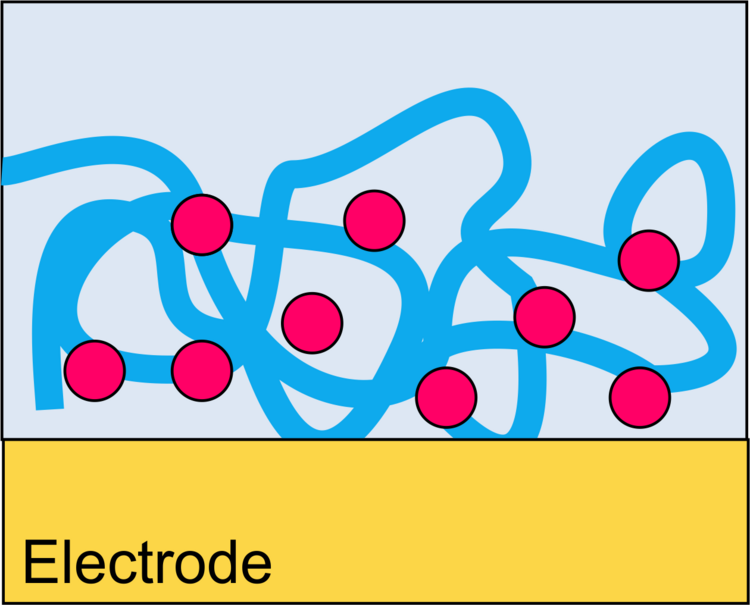
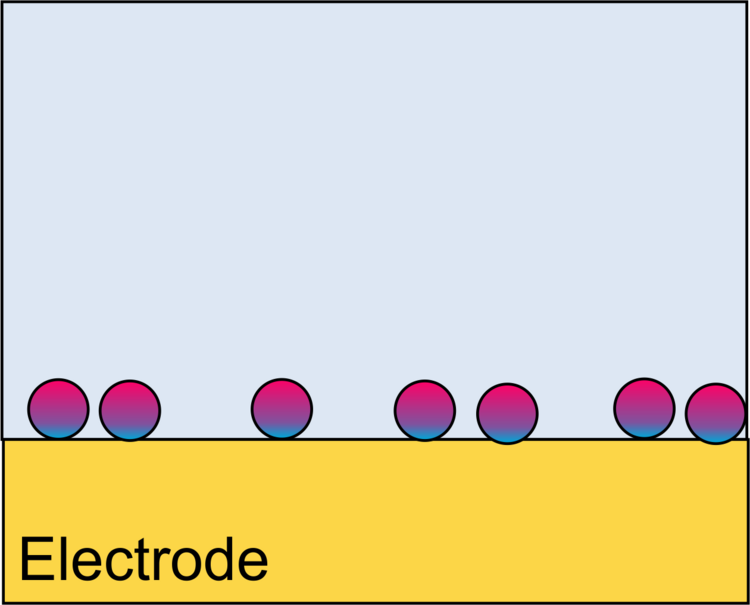

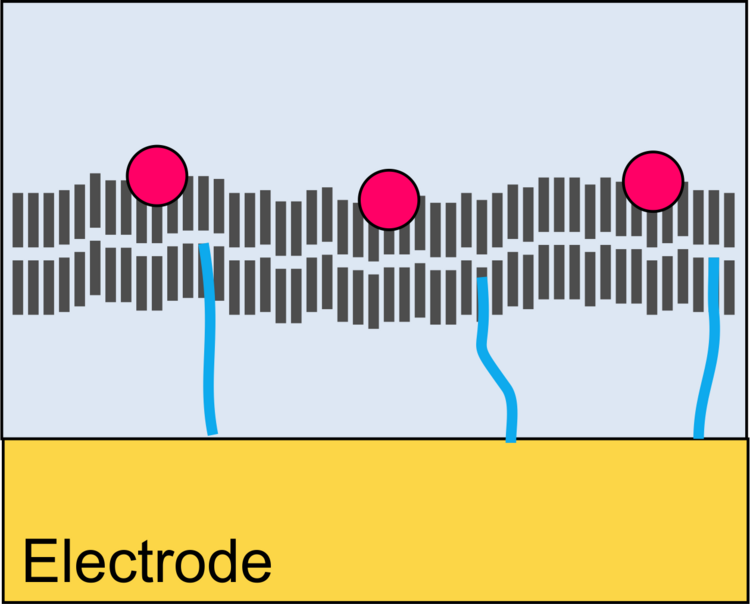
Reactivity Imaging of Immobilized Enzymes with the Scanning Electrochemical Microscope
Imaging of the activity of locally immobilized enzymes on surfaces can be performed with scanning electrochemical microscopy (SECM) in different ways.
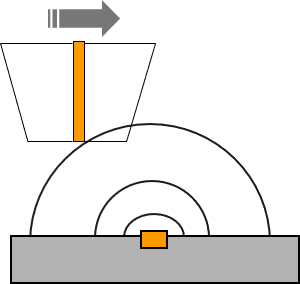
Imaging in Substrate-Generation/Tip Collection Mode
In this case, a redox active product formed by the enzyme is oxidized or reduced at the microelectrode of the SECM (Figure 13). The advantage of the method is a very high sensitivity and the possibility to measure not only oxidoreductases but also the activity of other important enzymes such as alkaline phosphatase or galactosidase. The disadvantage is the low resolution of the method and specific requirements to make these measurements quantitative.
Example. Mapping the activity of galactosidase (Figure 14) takes advantage of the fact that p-aminopehnol can be oxidized at the detection potential. However, the parent compound cannot. Figure 15 shows a raster image of three modified surface areas with a different amount of the enzyme galactosidase. A curve fit to a cut line in Figure 15 can be used for quantitative evaluation.


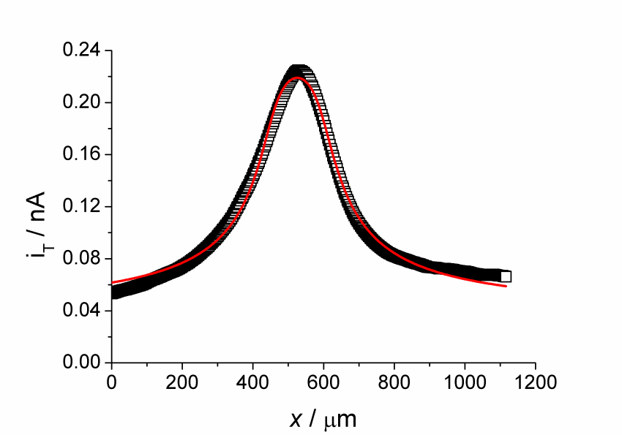
Imaging in Feedback Mode
In this case, the function of a natural cosubstrate of the enzyme (such as O2 for gluocose oxidase) is taken over by an artificial redox mediator (such as ferrocene derivatives for glucose oxidase). At the microelectrode of the scanning electrochemical microscope, the mediator (R in Figure 17) is continuously converted to its oxidized form. Current amplification occurs when the mediator is regenerated by the enzymatic reaction to be detected at the surface by the mediator accepting electrons from the enzyme.
The advantage of the method is the highly predictable quantitative relationship between the current at the microelectrode and that of the activity of the immbilized enzyme. The disadvantage is the lower sensitivity compared to the generator-collector mode.
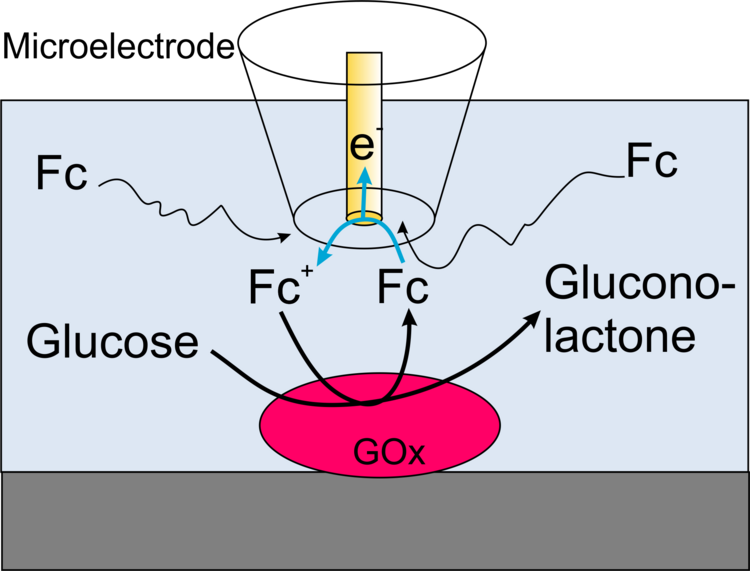
Example: In the schematic diagram of the coupled reactions in Figure 17, it can be seen that the oxidation of glucose at the enzyme glucose oxidase (Gox) only occurs when the ferrocene derivative oxidized at the microelectrode is available. In the enzymatic reaction, the original form of the mediator is regenerated and is again available for oxidation at the microelectrode, thus increasing the current. In the example measurement in Figure 18 and in the profile line, it can be seen that the edges are much sharper (higher lateral resolution) than in Figure 15 and 16 under roughly comparable conditions.
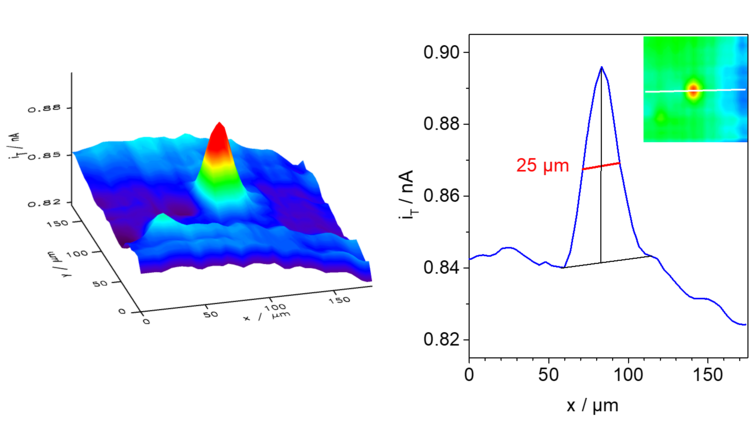
Imaging in redox competition mode
In cases where the reaction product of the enzymatic reaction is water, i.e., the solvent, the generator-collector mode cannot be used. In these cases, the redox competition mode can be applied.
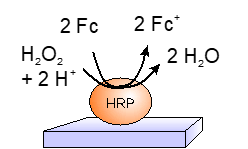
Example: The enzyme horseradish peroxidase (HRP) converts hydrogen peroxide H2O2 to water. The electrons can be supplied by an artificial electron donor (here ferrocene methanol, Fc, Figure 19). The activity of the enzyme can be measured either in generation-collection mode by detecting the oxidized form of ferrocene (ferrocenium, Fc+, Figure 20). This results in a reduction current at the microelectrode (plotted donwards). Another variant is the use of the redox-competition mode (Figure 21). Here the enzyme at the substrate and the microelectrode compete for the co-substrate ferrocene (Fc). The lower the local current, the more Fc is consumed by the enzyme. Figures 20 and 21 show approach curves. These are plots of the current at the microelectrode versus the distance between microelectrode and sample. The steeper the curve, the better the resulting resolution in two-dimensional images. Approach curves can also be used very well for mechanistic studies.
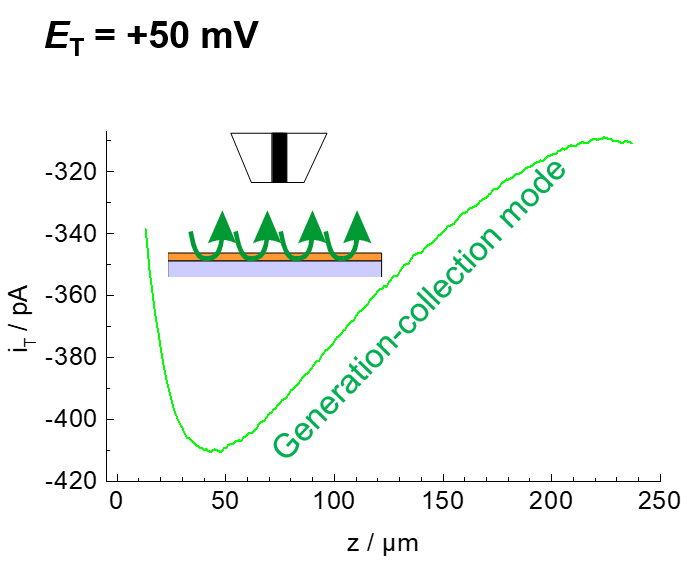
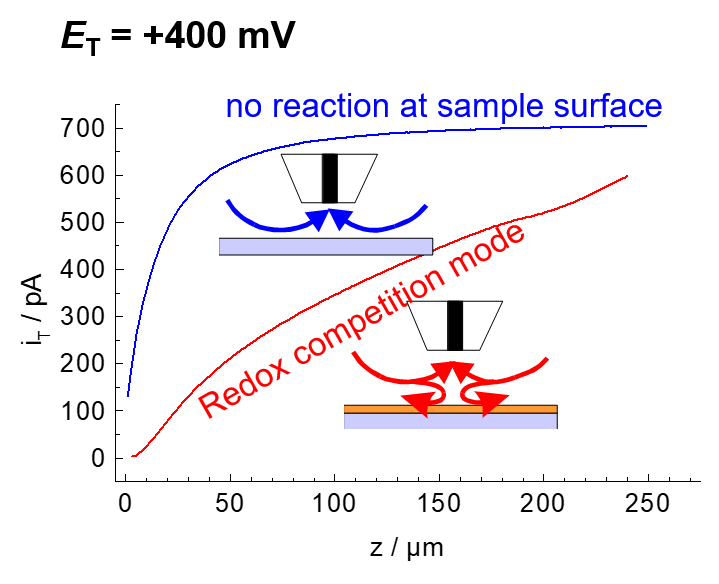
Own Contributions to the Area
Review Paper and Book Chapters
- B. R. Horrocks, G. Wittstock
Biotechnological Applications in Scanning Electrochemical Microscopy
in Scanning Electrochemical Microscopy, 3rd ed. (A. J. Bard, M. V. Mirkin, Eds.), CRC Press, Boca Raton 2022, Chap. 10, pp. 243-296. Abstract & Link - G. Wittstock, M. Burchardt, S. E. Pust, Y. Shen, C. Zhao
Scanning Electrochemical Microscopy for Direct Imaging of Reaction Rates
Angew. Chem. Int. Ed. 2007, 46, 1584-1617. Abstract & Link
- T. Wilhelm, G. Wittstock
Analysis of Interaction in Patterned Multienzyme Layers by Using Scanning Electrochemical Microscopy
Angew. Chem. Int. Ed. 2003, 42, 2247-2250. Abstract & Link - T. Wilhelm, G. Wittstock
Analyse von Wechselwirkungen in gemusterten Multi-Enzymschichten mit elektrochemischer Rastermikroskopie (SECM)
Angew. Chem. 2003, 115, 2350-2353. Abstract & Link - G. Wittstock
Modification and characterization of artificially patterned enzymatically active surfaces by scanning electrochemical microscopy
Fresenius J. Anal. Chem. 2001, 370, 303-315. Abstract & Link
Study of Systems with Two Interacting Emzymes
- M. Burchardt, G. Wittstock,
Micropatterned Multienzyme Devices with Adjustable Amounts of Immobilized Enzymes,
Langmuir 2013, 29 (48), 15090-15099, Abstract & Link - C. Zhao, G. Wittstock
An SECM detection scheme with improved sensitivity and lateral resolution: Detection iof galactosidase activity with signal amplification by glucose dehydrogenase
Angew. Chem. Int. Ed. 2004, 43, 4170-4172. Abstract & Link - C. Zhao, G. Wittstock
Ein SECM-Detektionsmodus mit verbesserter Empfindlichkeit und lateraler Auflösung: Detektion von Galactosidaseactivität mit Signalverstärkung durch Glucosedehydrogenase
Angew. Chem. 2004, 116, 4264-4267. Abstract & Link - G. Wittstock, W. Schuhmann
Formation and Imaging of Microscopic Enzymatically Active Spots on an Alkanethiolate-Covered Gold Electrode by Scanning Electrochemical Microscopy
Anal. Chem. 1997, 69, 5059-5066. Abstract & Link
Activity Imaging of Immobilized, Biotechnologically Relevant Enzymes
- Nogala, A. Celebanska, G.Wittstock, M.Opallo
Bioelectrocatalytic Carbon Ceramic Gas Electrode for Reduction of Dioxygen and Its Application in a Zinc–Dioxygen Cell
Fuel Cells 2010, 6, 1157-1163. Abstract & Link - Nogala, K. Szot, M. Burchardt, F. Roelfs, J. Rogalski, M. Opallo, G. Wittstock
Feedback mode SECM study of laccase and bilirubin oxidase immobilised in a sol–gel processed silicate film
Analyst 2010, 135, 2051–2058. Abstract & Link - Nogala, A. Celebanska, K. Szot, G. Wittstock, M. Opallo
Bioelectrocatalytic mediatorless dioxygen reduction at carbon ceramic electrodes modified with bilirubin oxidase
Electrochim. Acta 2010, 55, 5719–5724. Abstract & Link - W. Nogala, K. Szot, M. Burchardt, M. Joensson-Niedziolka, J. Rogalski, G. Wittstock, M. Opallo
Scanning electrochemical microscopy activity mapping of electrodes modified with laccase encapsulated in sol–gel processed matrix
Bioelectrochemistry 2010, 79, 101-107. Abstract & Link - C. Nunes Kirchner, M. Träuble, G. Wittstock
Study of Diffusion and Reaction in Microbead Agglomerates
Anal. Chem. 2010, 82, 2626-2635. Abstract & Link - M. Burchardt, M. Träuble, G. Wittstock
Digital Simulation of Scanning Electrochemical Microscopy Approach Curves to Enzyme Films with Michaelis-Menten Kinetics
Anal. Chem. 2009, 81, 4857–4863. Abstract & Link - P. C. Chen, R. L. C. Chen, T. J. Cheng, G. Wittstock
Localized Deposition of Chitosan as Matrix for Enzyme Immobilization
Electroanalysis 2009, 21, 804-810. Abstract & Link - K. Szot, W. Nogala, J. Niedziolka-Jönssona, M. Jönsson-Niedziolka, F. Marken, J. Rogalski, C. Nunes Kirchner, G. Wittstock, M. Opallo
Hydrophilic carbon nanoparticle-laccase thin film electrode for mediatorless dioxygen reduction SECM activity mapping and application in zinc-dioxygen battery
Electrochim. Acta 2009, 54, 4620–4625. Abstract & Link - M. J. W. Ludden, J. K. Sinha, G. Wittstock, D. N. Reinhoudt, J. Huskens
Control over binding stoichiometry and specificity in the supramolecular immobilization of cytochrome c on a molecular printboard
Org. Biomol. Chem. 2008, 6, 1553-1557. Abstract & Link - W. Nogala, M. Burchardt, J. Rogalski, M. Opallo, G. Wittstock
Scanning electrochemical microscopy study of laccase within a sol-gel processed silicate film
Bioelectrochemistry 2008, 72, 174-182. Abstract & Link - M. Burchardt, G. Wittstock
Kinetic studies of glucose oxidase in polyelectrolyte multilayer films by means of scanning electrochemical microscopy (SECM)
Bioelectrochemistry 2008, 72, 66-76. Abstract & Link - C. Nunes Kirchner, S. Szunerits, G. Wittstock
Scanning electrochemical microscopy (SECM) based detection of oligonucleotide hybridization and simultaneous determination of the surface concentration of immobilized oligonucleotides on gold
Electroanalysis 2007, 19, 1258-1267. Abstract & Link - C. Nunes Kirchner, C. Zhao, G. Wittstock
Analysis of the activity of beta-galactosidase from Escherichia coli by scanning electrochemical microscopy (SECM)
Comprehensive Analytical Chemistry 2007, 49, e371-e379. Abstract & Link - C. Nunes Kirchner, G. Wittstock
Kinetic analysis of titanium nitride thin films by scanning electrochemical microscopy
Comprehensive Analytical Chemistry 2007, 49, e363-e370. Abstract & Link - G. Wittstock, M. Burchardt, C. Nunes Kirchner
Scanning electrochemical microscopy in biosensor research
Comprehensive Analytical Chemistry 2007, 49, 907-939. Abstract & Link - M. Zhang, G. Wittstock, Y. Shao, H. H. Girault
Scanning Electrochemical Microscopy as a Readout Tool for Protein Electrophoresis
Anal. Chem. 2007, 79, 4833-4839. Abstract & Link - O. Sklyar, M. Träuble, C. Zhao, G. Wittstock
Modeling Steady-State Experiments with a Scanning Electrochemical Microscope Involving Several Independent Diffusing Species Using the Boundary Element Method
J. Phys. Chem. B 2006, 110, 15869-15877. Abstract & Link - C. Zhao, G. Wittstock
Scanning electrochemical microscopy for detection of Biosensor and biochip surfaces with immobilized pyrroloquinone (PQQ)-dependent glucose dehydrogenase as enzyme label
Biosens. Bioelectron. 2005, 20, 1277-1284. Abstract & Link - C. Zhao, J. Sinha, C. A. Wijayawardhana, G. Wittstock
Monitoring beta-Galactosidase Activity by Means of Scanning Electrochemical Microscopy
J. Electroanal. Chem. 2004, 561, 83-91. Abstract & Link - C. Zhao, G. Wittstock
Scanning Electrochemical Microscopy of Quinoprotein Glucose Dehydrogenase
Anal. Chem. 2004, 76, 3145-3154. Abstract & Link - T. Wilhelm, G. Wittstock
Generation of Periodic Enzyme Patterns by Soft Lithography and Activity Imaging by Scanning Electrochemical Microscopy.
Langmuir 2002, 18, 9486-9493. Abstract & Link - G. Wittstock, T. Wilhelm
Characterization and Manipulation of Microscopic Biochemically Active Regions by Scanning Electrochemical Microscopy (SECM)
Anal. Sci. 2002, 18, 1199-1204. Abstract & Link - T. Wilhelm, G. Wittstock
Patterns of functional proteins formed by local electrochemical desorption of self-assembled monolayers
Electrochim. Acta 2001, 47, 275-281. Abstract & Link - G. Wittstock, T. Wilhelm, S. Bahrs, P. Steinrücke
SECM feedback imaging of enzymatic activity on agglomerated microbeads
Electroanalysis 2001, 13, 669-675. Abstract & Link - C. A. Wijayawardhana, N. J. Ronkainen-Matsuno, S. M. Farrel, G. Wittstock, H. B. Halsall, W. R. Heineman
Microspot Enzyme Assays with Scanning Electrochemical Microscopy
Anal. Sci. 2001, 17 Supplement, 535-538. Abstract & Link - J. Zaumseil, G. Wittstock, S. Bahrs, P. Steinrücke
Imaging the activity of nitrate reductase by means of a scanning electrochemical microscope
Fresenius J. Anal. Chem. 2000, 367, 346-351. Abstract & Link - C. A. Wijayawardhana, G. Wittstock, H. B. Halsall, W. R. Heineman
Electrochemical Immunoassay with Microscopic Immunomagnetic Bead Domains and Scanning Electrochemical Microscopy
Electroanalysis 2000, 12, 640-644. Abstract & Link - C.A. Wijayawardhana, G. Wittstock, H.B. Halsall, W.R. Heineman
Spatially Addressed Deposition and Imaging of Biochemically Active Bead Microstructures by Scanning Electrochemical Microscopy
Anal. Chem. 2000, 72, 333-338. Abstract & Link - T. Wilhelm, G. Wittstock, R. Szargan
Scanning electrochemical microscopy of enzymes immobilized on structured glass-gold substrates
Fresenius J. Anal. Chem. 1999, 365, 163- 167. Abstract & Link - C. Kranz, G. Wittstock, H. Wohlschläger, W. Schuhmann
Imaging of Microstructured Biochemically Active Surfaces with Scanning Electrochemical Microscopy
Electrochim. Acta 1997, 42, 3105-3111. Abstract & Link - G. Wittstock, R. Hesse, W. Schuhmann
Patterned Self-Assembled Alkanethiolate Monolayers on Gold. Patterning and Imaging by Means of Scanning Electrochemical Microscopy
Electroanalysis 1997, 9, 746-750. Abstract & Link - G. Wittstock, K. Yu, H. B. Halsall, T. H. Ridgway, W. R. Heineman
Imaging of Immobilized Antibody Layers with Scanning Electrochemical Microscopy
Anal. Chem. 1995, 67, 3578-3582. Abstract & Link