Contact
Mailing Address
Visitors
Oxygen Electrodes for Metal-Air Batteries
by Marius Muhle
Lithium-ion batteries are characterized by a very high energy density and high efficiency, which is why they are essential for the energy and mobility transition. The positive electrodes of lithium-ion batteries require transition metals such as Co or Ni, which are in limited supply and cause environmental damage when mined. The positive electrode is needed to oxidize the negative electrode during battery discharge. Oxidation processes are known from everyday life mainly as reactions with atmospheric oxygen. The reaction of oxygen with lithium as a promising material for negative electrodes could be used in a lithium-air battery. The reaction equation is as follows:

Due to its low price and non-toxicity, oxygen could be a good reaction partner at the positive electrode. The extremely high energy density of lithium-air batteries also makes their development a promising field of research.[1]
However, the use of oxygen also presents a technical and chemical challenge. In order to enter into the desired reaction, the gaseous oxygen must be dissolved in the battery electrolyte and have contact with an electrical conductor that provides/dissipates the electrons. This is achieved using a gas diffusion electrode (GDE), which conducts electrically, allowing gaseous oxygen to pass into the battery but preventing electrolyte leakage. Figure 2 shows the structure of a lithium-air battery.
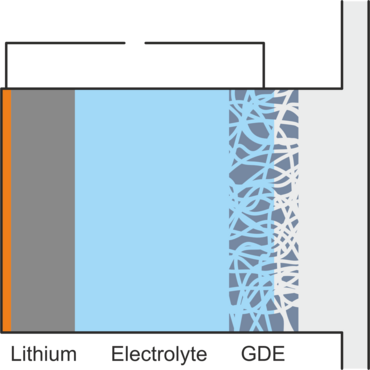
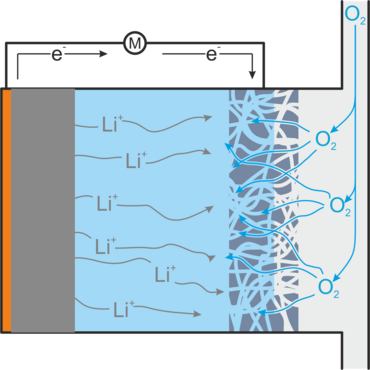
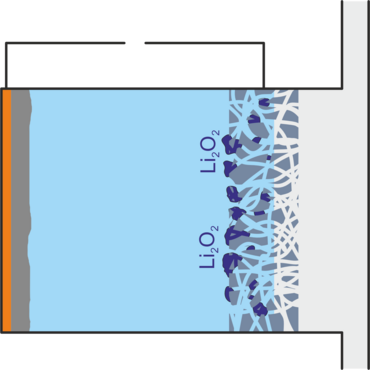
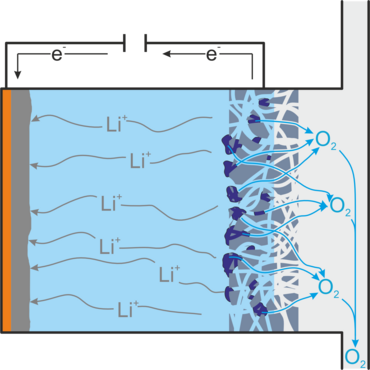
When the battery is discharged, lithium peroxide is formed, which is deposited as a solid in the gas diffusion electrode and can thus clog it. Highly reactive superoxide is also formed, which decomposes the electrolyte, and inert lithium oxide, which is not accessible for a reverse reaction. Both reduce the service life of the battery. Other unwanted side reactions occur when impurities such as water and carbon dioxide enter the battery, as would be the case if air were used directly as an oxygen source. In addition, lithium peroxide particles may lose contact with the gas diffusion electrode and thus no longer be accessible for the charging reaction.[1,2]
A possible solution to this problem investigated in our group is the use of redox mediators in the electrolyte. The compounds transport the electrons between the gas diffusion electrode and the lithium peroxide like a ferry. In this way, the electrons required for the reaction can be transferred to the lithium peroxide without the latter having to be in electronic contact with the gas diffusion electrode.[3]
To advance the development of lithium-air technology, an even better understanding of the processes at the gas diffusion electrode is required. Using different variants of scanning electrochemical microscopy (SECM), the local conductivity and oxygen permeability of the gas diffusion electrode are measured in our research group as a function of the state of charge of the cell. To measure the oxygen permeability, an oxygen-containing gas mixture flows on one side of the gas diffusion electrode. On the other side of the electrode is a microelectrode in an electrolyte solution, at which the reaction of oxygen species in solution can be detected.[4-6] Figure 3 shows such a measurement setup. Different oxygen species can also be detected by electrochemical scanning microscopy and additionally by X-ray photoelectron spectroscopy.
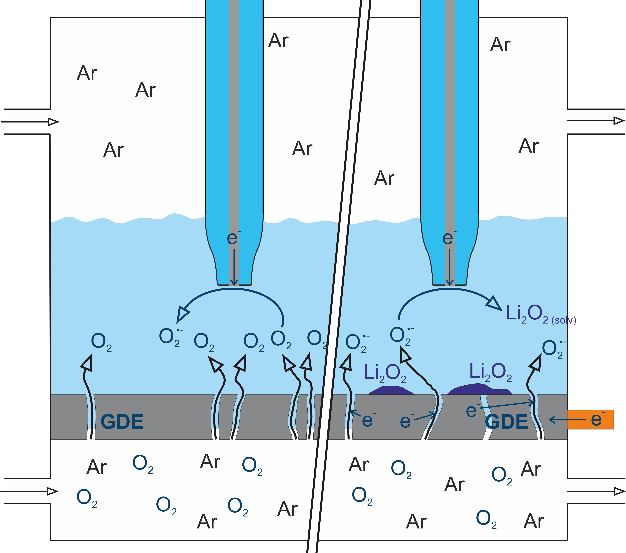
References
[1] W.-J. Kwak, Rosy, D. Sharon, C. Xia, H. Kim, L. R. Johnson, P. G. Bruce, L. F. Nazar, Y.-K. Sun, A. A. Frimer, M. Noked, S. A. Freunberger, D. Aurbach; Lithium-Oxygen Batteries and Related Systems: Potential, Status, and Future. Chem. Rev. 2020, 120, 6626–6683. https://doi.org/10.1021/acs.chemrev.9b00609
[2] R. Padbury, X. Zhang; Lithium–oxygen batteries—Limiting factors that affect performance. J. Power Sources 2011, 196, 4436–4444. https://doi.org/10.1016/j.jpowsour.2011.01.032
Own Contribution to the Research Field
[3] B. Krueger, K. K. Rücker, G. Wittstock
Redox Mediators for Faster Lithium Peroxide Oxidation in a Lithium-Oxygen Cell: A Scanning Electrochemical Microscopy Study
ACS Appl. Energy Mater. 2022, 5, 3724-3733. Abstract & Link
[4] P. Schwager, D. Fenske, G. Wittstock
Scanning electrochemical microscopy of oxygen permeation through air-electrodes in lithium–air batteries
J. Electroanal. Chem. 2015, 740, 82-87, Abstract & Link
[5] H. Bülter, P. Schwager, D. Fenske, G. Wittstock
Observation of Dynamic Interfacial Layers in Li-Ion and Li-O2 Batteries by Scanning Electrochemical Microscopy
Electrochim. Acta 2016, 199, 366-379. Abstract & Link
[6] P. Schwager, S. Dongmo, D. Fenske, G. Wittstock
Reactive oxygen species formed in organic lithium-oxygen batteries
Phys. Chem. Chem. Phys. 2016, 18, 10774-10780, Abstrac & Link