Contact
Mailing Address
Visitors
Spectroelectrochemistry of Model Biomembranes
Izabella Brand
Biomembranes are the most important charged interfaces in nature. They are composed of a lipid bilayer and membrane-associated proteins. Membranes are constantly involved in interactions with ions, small organic molecules as well as with biomacromolecules present on both sides of the membrane. Der are very strong electric field across the membrane caused by the unequal distribution of ions on both side of the biomembrane. Model membrane on electrodes are able to mimic the effects of electric fields on the arrangement of the molecular components within a biomembrane. Proteins belong to the most important class of macromolecules interacting with biological membranes. In-situ structural studies of the protein conformation and orientation at membranes are extremally challenging. Polarization modulation infrared reflection absorption spectroscopy (PM IRRAS) provides the possibility to probe in situ conformational changes in proteins interacting with the model membranes. Proteins developed different modes of interaction and association to the lipid membrane, as illustrated in Figure 1. These different interaction modes are accompanied by changes in the protein conformation, secondary structure, and hydration compared to the protein structure in the solution phase.
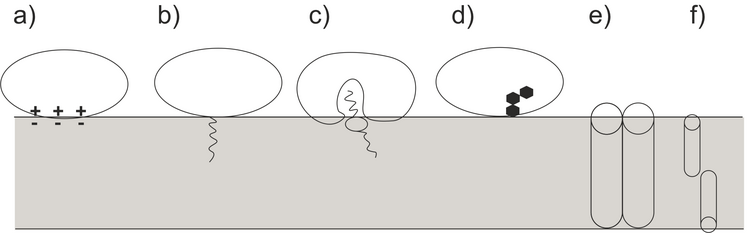
Figure 2 shows an example from our current studies of the lipid-anchor protein, specifically the association of the protein recoverin to the membrane at different polarization states. Recoverin is a cytoplasmic protein found in photoreceptor cells. This protein contains a myristoyl (C14) chain. In the presence of Ca2+, the C14 is inserted into the hydrophobic fragment of a lipid bilayer membrane. Figure 2 shows PM IRRA spectra in the region of the amide I’ band of recoverin when associated to a lipid bilayer at different potentials applied to the Au electrode, onto which the bilayer is prepared. Using second derivative of the measured spectra (lower panels in figure 2), the amide I’ mode was deconvoluted into two modes associated with disordered fragments (1670 cm-1, red) and a-helices (1648 cm-1, blue).
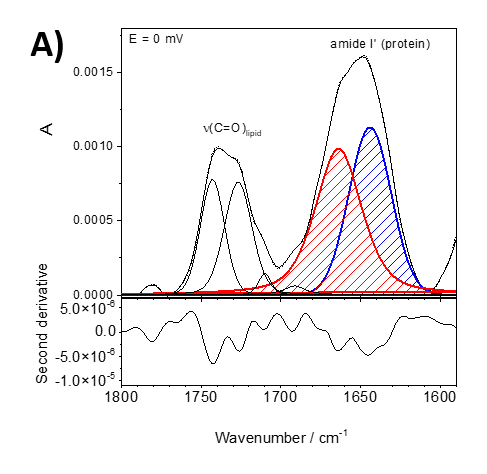
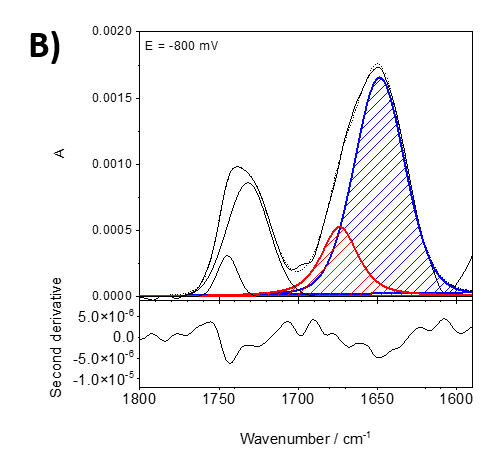
Figure 2. Deconvoluted in-situ PM IRRA spectra (upper panel) and their second derivative (lower panel) in the 1800 – 1580 cm-1 spectral region of the DMPC:cholesterol lipid bilayer after interaction with recoverin deposited on the Au surface. A) bei 0 mV, B) bei -800 mV.
Electric potentials have a large impact on the shape and intensity of the amide I’ band, indicating potential-driven changes in the protein conformation and orientation. These changes are reversible in potential scans.
PM IRRAS is sensitive to the orientation of a particular molecular fragment in an anisotropic film, such as a model cell membrane. According to the surface selection rule of IRRAS, the intensity of an IR absorption mode in an anisotropic film depends on the surface concentration of species adsorbed on a solid surface and on theangle between the transition dipole moment vs. the surface normal. Knowing the direction of the transition dipole moment within a studied molecule, the angle between this vector and the surface normal can be determined. We performed these analyses for the amide I’ of the helical conformation to find the average orientation of the helices in the membrane associated state. These results are shown in Figure 3.
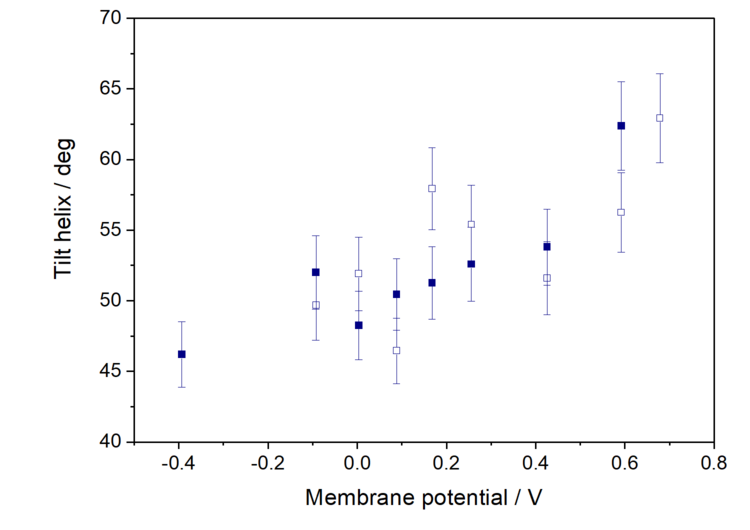
At positive membrane potentials, the helices lie preferentially parallel to the membrane surface and a negative potential shift causes a gradual reorientation of the protein leading to the removal of the myristoyl chain. This is connected with reorientation of the lipid molecules at negative potentials.
This example illustrates the applicability of in-situ PM IRRAS to study structural alterations in supramolecular assemblies at a sub-molecular level. These studies are not limited to models of biomembranes, but have been extended to the study of:
- Electrical double layer of ionic liquids
- Potential-driven changes in monolayers of redox-active metallosurfactants with rectifying properties
- Potential-driven changes in conformation and stability of DNA double helices, their interaction with small zwitterionic molecules and enzymes
- Redox-active polymer films
- Potential-dependent misfolding of protein films
- Corrosion of light metals such as magnesium or aluminum.